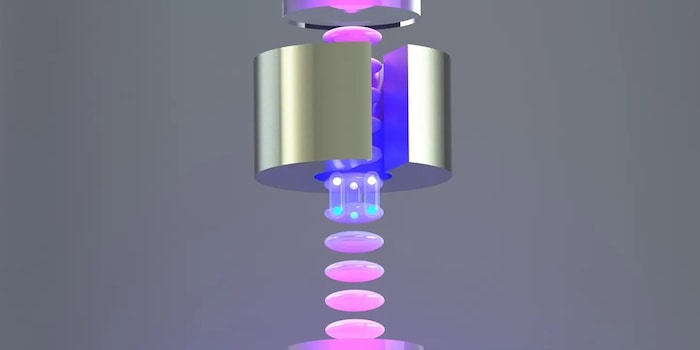
Physicists use atoms to measure gravity with extreme precision

The accuracy of their unusual experimental set-up significantly exceeds that of previous experiments. It can also be used to test hypotheses about dark energy.
It is questionable whether Isaac Newton really dropped an apple on his head and thus came up with the theory of gravity. What is undisputed, however, is that he realised sometime around 1665 that objects attract each other. In the meantime, the phenomenon of gravity is well known and the associated theories have been well researched. However, although gravity is the predominant force in nature on large scales, it is difficult to measure experimentally. Many questions remain unanswered, such as how it behaves on small scales or what role it plays in effects attributed to dark matter and dark energy. Physicists at the University of California at Berkeley have now set up the most precise experiment to date to search for minor deviations from the recognised theory of gravity. They report on this in the scientific journal "Nature".
For the experiment, the research team combined an atom interferometer with an optical lattice. An atomic interferometer is a special measuring device that utilises the wave properties of atoms to determine the gravitational constant with high accuracy. An optical lattice is a spatially periodic structure consisting of two crossed laser beams in which atoms or molecules can be trapped. The laser light generates an electric dipole moment in each of the atoms, causing the atom to experience a force from the electromagnetic radiation. The combination of atomic interferometer and optical grating enables the researchers to capture caesium atoms for several seconds instead of milliseconds. This allows gravitational effects to be analysed even better than before - by a factor of three to five, the team writes.
However, although they were initially unable to find any deviation from the predictions of the theory put forward by Isaac Newton 400 years ago, the significantly improved precision may help, for example, to investigate the force of gravity at the quantum level, says Holger Müller, professor of physics at UC Berkeley, according to a press release from his university. While physicists already have good evidence of the quantum nature of three of the four forces of nature - electromagnetism and the strong and weak interactions - the quantum nature of the gravitational force has not yet been proven.
Experimental proof of the quantum nature of gravity is lacking
"Most theorists probably agree that gravity is quantum. But no one has ever seen any experimental proof of this," says Müller. "But because the sensitivity of our experiment increases exponentially with the holding time of the atoms, we have an increased chance of finding experimental proof."
And the research team has another hope: they want to use their experiment to track down dark energy, the mysterious force that is driving the universe apart ever faster. The realisation that the universe is expanding at an accelerated rate came more than 25 years ago from the observation of supernovae, which are all moving away from us - and at an ever-increasing rate. One of many proposals to explain this observation is that dark energy is a fifth, repulsive fundamental force, much weaker than gravity and mediated by a hypothetical particle called a "chameleon". In the emptiness of space, this particle could push space apart. In a laboratory on Earth, however, where it is shielded from matter, it would have an extremely short range. However, this particle has not yet been discovered.
The experimental setup can be used to measure very fine gravitational effects because each atom can be placed in a spatial superposition of two quantum physical states. This means that the individual atoms are in two places at the same time, so to speak. If they are brought close to a tungsten weight, the state that is closer to the weight experiences a stronger attractive force, which changes the phase of the atom. As soon as the wave function of the atom collapses, the phase difference shows the difference in gravitational attraction between them. This allows even the smallest discrepancies to be resolved, which would be lost in other measurements.
Atomic interferometry is the art of utilising the quantum properties of a particle, i.e. the fact that it behaves both like a particle and like a wave.
"Atomic interferometry is the art of utilising the quantum properties of a particle, i.e. the fact that it behaves like both a particle and a wave. We split the wave so that the particle travels two paths simultaneously and then superimpose them again at the end," explains Müller. "The waves can either be in phase and add up, or they can be out of phase and cancel each other out. The trick is that the phase depends very sensitively on some of the variables you want to measure, such as acceleration, gravity, rotation or fundamental constants."
If the predicted chameleon particles do exist, then this will manifest itself in the way the atoms are attracted to the tungsten weight. There should therefore be small deviations from the usual theories of gravity. As the experimenters were unable to recognise any such deviation, they were able to significantly narrow down the energy range in which one can search for chameleon particles. In the meantime, the physicists are already working on improving the lattice atom interferometer even further to make it sensitive enough to detect the quantum properties of gravity - and thus answer one of the most pressing questions in the field.
Spectrum of Science
We are partners of Spektrum der Wissenschaft and want to make well-founded information more accessible to you. Follow Spektrum der Wissenschaft if you like the articles.
Original article on Spektrum.de

Experts from science and research report on the latest findings in their fields – competent, authentic and comprehensible.